Brain Development
The journey of how the human brain develops from the moment of fertilization until a child is a few years old is amazing. It starts with a tiny cell that grows and divides, eventually forming the brain, one of the most complex parts of the human body capable of thinking, feeling, and understanding.
This story of growth begins when two genetic materials come together and continues as these cells multiply and organize into what becomes our brain. This process is like a well-planned dance where each step is important for creating a fully working brain. Early on, cells decide whether they will become neurons (the cells that send messages in the brain) or glial cells (the cells that support and protect neurons). They move to the right places following a set of genetic instructions,
which is crucial for the brain to work properly.
In this note, we're going to look closely at how the human brain grows from the very beginning until a baby grows into a young child. We'll talk about how neurons are created, how they connect with each other to let us see, move, and learn, and how important the first years of life are for setting up these connections. Understanding how the brain develops helps us see how amazing life is and shows why it's so important to take care of young children's environments to help their
brains grow well.
We'll go through each step of how the brain forms, the obstacles it overcomes to build networks that let us do everything from grabbing objects to remembering our names, and how adaptable a young child's brain is. From the start, the development of the brain is a story about how strong and complex humans are, showing the incredible things that are part of our biology.
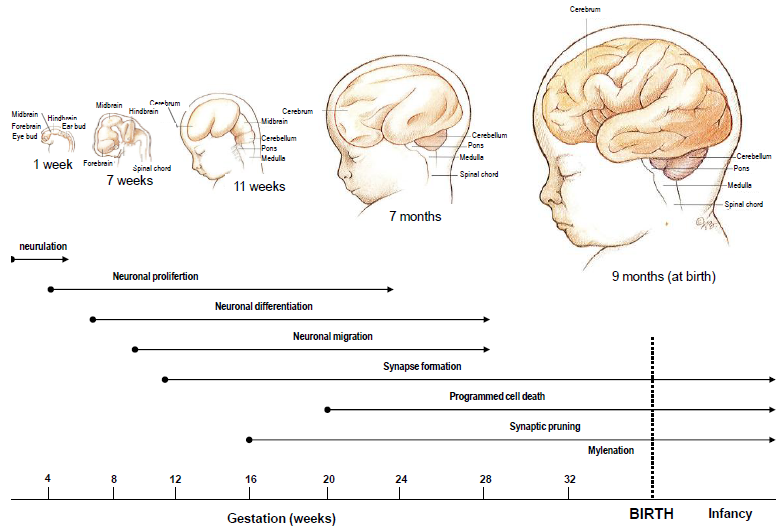
Source : The first five years: Starting early. Early Childhood Series No. 2. 2011
Major Stages
Let's take a closer look at the key steps in how the brain grows and changes from the very beginning of life until a child is a few years old. We will discover how the brain develops from just a few cells to a complex network that lets us think, feel, and learn
NOTE : Before you reading the details, I would suggest you to watch this video(Prenatal Brain Development and Pregnancy - How a Wonder is Born! (Animation) ) first and try to be able to visualize the progress of the brain shaping in your mind.
Neurulation (Approximately 3-4 weeks gestation)
This is the first stage shown on the timeline and involves the formation of the neural tube, which will eventually develop into the central nervous system, comprising the brain and spinal cord.
Here are more details about neurulation, broken down into bullet points for clarity:
-
Start of Neurulation: Around 3-4 weeks after a baby is conceived, a key process called neurulation begins.
-
Formation of Neural Plate: The cells in the developing embryo organize into a flat structure known as the neural plate.
-
Folding of the Neural Plate: This plate then starts to fold inwards, forming a hollow tube. This is the neural tube.
-
Closure of the Neural Tube: The neural tube closes from the middle outwards, sealing like a zipper from the bottom end to the top end.
-
Development into Central Nervous System: The neural tube will become the central nervous system, which is made up of the brain and the spinal cord.
-
Division into Brain Sections: As the tube closes and forms, it will eventually divide into sections that will develop into different parts of the brain.
-
Spinal Cord Formation: The part of the neural tube that does not become the brain will extend to form the spinal cord.
- Foundation for Future Growth: This stage sets the foundation for all the complex growth that will follow as the baby develops.
Neuronal proliferation (Approximately 5-20 weeks gestation)
This stage involves the rapid creation of neurons. It's a phase of extensive cell division, during which the number of neurons increases dramatically.
-
Start of Neuronal Proliferation: Begins around the 5th week after conception.
-
Rapid Division of Cells: Neural stem and progenitor cells divide rapidly, marking the start of extensive neuron formation.
-
Exponential Growth of Neurons: There's an exponential increase in the number of neurons, from fewer than 100,000 initially to over 100 billion by the end of the proliferation stage.
-
Peak Production Rates: By week 5, the brain produces more than 2,500 neurons each minute.
-
Short Cell Cycle: During the peak of proliferation, the cell cycle length for neural stem cells can be less than 12 hours.
-
Primary Zones of Activity: Most neuron formation occurs in the ventricular and subventricular zones of the brain.
-
Migration of New Neurons: After being created, neurons migrate outward to their designated layers in the cortex.
-
Development of Complex Brain Functions: Neurons in the frontal lobe, which are responsible for complex functions, develop later in the process.
-
Synapse Formation Surge: There is a significant increase in synapse formation between neurons, facilitating communication.
-
Role of the Subplate Layer: This layer acts as a temporary staging area for new neurons during development.
-
Apoptosis and Pruning: Apoptosis, or programmed cell death, helps refine the circuits by eliminating excess neurons, a process known as neural pruning.
-
Timed Neurogenesis Waves: The creation of neurons happens in timed waves across different brain regions, ensuring organized development.
-
Cortical Neuron Numbers: By the 10th week, the cortex already has billions of neurons.
-
Glial Cell Multiplication: Alongside neurons, glial cells also proliferate to provide support and maintain neuron health.
-
Foundation for Neural Networks: The dramatic increase in neurons allows for the formation of complex neural networks.
-
Cytoskeleton Dynamics: The structural development of neurons is managed by the dynamics of the cellular cytoskeleton.
-
Importance of Cell Cycle Regulation: Proper regulation of the cell cycle is crucial to prevent developmental abnormalities.
-
Balance in Brain Development: There is a need for tight control over differentiation (specialization of cells), migration (movement to the right place), and connectivity (forming the right links).
-
Intelligence Foundation: The rapid and precise pattern of neuron production lays the foundation for intelligence and brain function.
Neuronal differentiation (Throughout gestation)
After neurons are created, they begin to differentiate, meaning they start to take on specialized functions. This is when neurons develop distinct characteristics to become different types of cells within the brain.
-
Migration to Destinations: Neurons move from their birthplaces in germinal zones to their final locations in the brain.
-
Influence of Chemical Signals: External chemical signals play a crucial role in determining the specific identities and functions of differentiating neurons.
-
Morphological Specialization: Neurons develop specialized shapes and structures depending on their location and the connections they form.
-
Purpose and Integration: Differentiation sets the stage for each neuron's future function and its integration into brain circuits.
-
Order of Neuron Creation: Excitatory projection neurons, which activate other neurons, are created first, followed by inhibitory neurons that help regulate brain activity.
-
Developmental Sequence in Brain Regions: The brain's occipital lobe, responsible for visual processing, develops its reception areas before the frontal lobe, which is crucial for complex cognitive tasks.
-
Priority of Sensory Pathways: Sensory systems, such as the auditory pathway, develop earlier than regions responsible for higher consciousness.
-
Early Maturation of Motor Neurons: Neurons involved in movement mature early to support the development of motor skills.
-
Role of Synaptic Connectivity: The formation and maintenance of synapses influence the final structure and function of neurons.
-
Neuronal Types Based on Neurotransmitters: Glutamatergic neurons use glutamate for excitation, while GABAergic neurons use GABA for inhibition.
-
Support from Astrocytes: Agranular astrocytes play a supportive role, affecting synapse function and neuronal health.
-
Myelination by Oligodendrocytes: Oligodendrocytes, appearing later in development, are responsible for myelinating neurons in the cortex, enhancing signal transmission.
-
Expression of Molecular Components: Ion channels, receptors, and transporters express differently across neurons, tailored to their specific functions.
-
Signaling for Network Coordination: Calcium wave signaling among neurons coordinates early network activities and connections.
-
Epigenetic Regulation: Genetic programs that drive neuronal differentiation are regulated by epigenetic mechanisms, ensuring proper development.
-
Guidance for Neuron Migration: Both short and long-distance cues guide neurons to their correct destinations during migration.
-
Specialization in Later Stages: As development progresses, neurons become increasingly specialized for specific tasks.
-
Membrane Potential and Maturity: Variations in membrane potentials among neurons indicate their functional maturity and readiness for activity.
-
Impact of Developmental Errors: Mistakes in differentiation and migration processes can lead to developmental disorders, including autism.
-
Integration into Circuits: Developing neurons are carefully integrated into existing brain circuits, enabling future sensory, motor, and cognitive functions.
Neuronal migration (Approximately 6-24 weeks gestation)
During this stage, neurons move from their birthplace to their final position in the brain. This migration is critical for the proper organization of the brain.
-
Commencement: Neuronal migration starts around the 6th week as neurons become postmitotic, ceasing to divide and beginning their journey to their final destinations.
-
Migration Pathways: Neurons migrate from the ventricular zone outward along radial glia, which act as scaffolding for this movement.
-
Pattern and Timing: Migration occurs in timed waves, continuing over weeks and months, with cortical layers forming in an inside-out pattern, meaning that later-born neurons layer over earlier ones.
-
Postnatal Development: The frontal and prefrontal regions of the brain, important for complex cognitive functions, continue their development after birth.
-
Mechanisms of Movement: Nucleokinesis, involving the translocation of the nucleus within the cell, and dynamic rearrangements of the cytoskeleton, are key drivers of neuron movement.
-
Long-Distance Travel: Neurons travel long distances from their origin sites to their final locations, guided by glial pathways.
-
Guidance Signals: Migration is directed by a mix of chemoattractant and chemorepulsive signals, ensuring cells move to the correct locations.
-
Migratory Modes: Cells adopt different modes of migration, such as multipolar and bipolar locomotion or terminal translocation, depending on the zone they are navigating.
-
Positioning and Connectivity: The final positioning of neurons is crucial for establishing local connections within the brain.
-
Migration Errors: Errors in migration can lead to ectopic (misplaced) neurons, which disrupt the brain's wiring and function.
-
Cortical Organization: The spatio-temporal order of migration is essential for proper cortical expansion and the layering of neurons.
-
Signal Regulation: Migration is regulated by signals from neurotransmitters like GABA and glutamate, as well as adhesion molecules, coordinating the flow of neurons.
-
Preplate and Subplate Dynamics: Early and late arriving neurons contribute to the splitting of the preplate, with the displacement of subplate neurons facilitating cortical maturation.
-
Orchestration and Timing: The precise timing of neuronal migration is critical for the subsequent functional integration of neurons into the brain's circuits.
-
Formation of Regional Networks: The alignment of neuronal layers is fundamental for the formation of regional networks within the brain, supporting various functions.
Synapse formation (Starts around mid-gestation and continues postnatally)
Synapses are the connections between neurons that allow them to communicate with each other. This period is marked by a significant increase in synapse formation, also known as synaptogenesis.
-
Initiation Timing: Synapse formation begins as early as weeks 16-17 of gestation, with neurons starting to establish connections.
-
Signal Transmission: Enables the transmission of signals between neurons, essential for all brain functions.
-
Types of Synapses: Includes both chemical synapses, using neurotransmitters like glutamate, and electrical synapses, which pass ions directly through gap channels.
-
Structural Components: Dendritic spines and axon terminals meet at synapses, with the presynaptic terminal releasing neurotransmitters detected by the postsynaptic side.
-
Promotion of Development: Neurotrophic factors aid in the structural development of synapses, while cell adhesion molecules ensure the connection of pre- and post-synaptic sites.
-
Rapid Increase: A significant rise in synapse formation occurs around mid-gestation, marking the onset of neural connectivity, with over 50 million new synapses forming per minute during the 3rd trimester.
-
Functional Differentiation: Excitatory projection neurons and inhibitory interneurons play distinct roles, linking sensory inputs to higher brain regions and modulating cortical activity, respectively.
-
Circuit Formation: Essential sensory pathways, like thalamocortical circuits, become established, enhancing brain connectivity.
-
Deployment of Synaptic Machinery: Includes the arrangement of vesicles and receptors essential for synaptic transmission.
-
Early Dynamics: Initial synapses exhibit high turnover rates, with activity-dependent processes stabilizing functional synapses influenced by sensory experiences.
-
Plasticity and Pruning: Glutamatergic NMDA receptors are crucial for mediating plasticity changes, with synaptic pruning removing less active connections.
-
Developmental Windows: Critical periods for sensory development begin, highlighting the importance of early experiences in shaping neural connections.
-
Postnatal Formation: Synapse formation continues exponentially after birth, contributing to the rapid development of the brain during infancy.
-
Regulation of Neural Flow: A balance between excitation and inhibition at synapses is vital for regulating neural signal flow, affecting learning and memory development.
Programmed cell death (Late gestation to postnatal)
This is a normal part of brain development where excess neurons and synapses are eliminated, a process known as apoptosis. It helps to refine the wiring of the brain.
-
Phase Timing: Apoptosis occurs from late gestation through the postnatal period, playing a key role in sculpting the brain's architecture.
-
Essential for Refinement: This natural process eliminates excess neurons and synapses, refining the brain's neural networks for efficient functioning.
-
Begins with Postmitotic Neurons: Programmed cell death starts as neurons stop dividing and start differentiating, highlighting its role in the later stages of brain development.
-
Mechanisms of Elimination: Cells destined for apoptosis are removed through a series of biochemical events, ensuring that only neurons with established connections survive.
-
Contribution to Neural Circuitry: By eliminating redundant pathways, apoptosis contributes to the specificity and efficiency of neural circuits.
-
Balance with Neurogenesis: Apoptosis is balanced with the processes of neuron formation and differentiation, ensuring the proper number of neurons is maintained.
-
Influenced by Neurotrophic Factors: Survival of neurons during this phase is often dependent on neurotrophic factors, which signal that a neuron has successfully formed functional connections.
-
Closely Linked with Synaptic Pruning: While apoptosis reduces neuron numbers, synaptic pruning refines connections between surviving neurons, both processes working together to optimize brain function.
-
Regulated by Genetic and Environmental Factors: Both genetic programming and environmental conditions influence the extent and timing of apoptosis, underlining the complex interplay between genes and the environment in brain development.
-
Critical for Healthy Brain Development: Proper execution of apoptosis is crucial for the development of a healthy, functional brain, with errors in this process linked to developmental disorders.
Synaptic pruning (Late infancy and beyond)
Following the initial overproduction of neurons and synapses, the brain begins to prune away the less active synapses. This is an important process of brain development that helps to strengthen the most important and frequently used neural connections.
-
Timeline and Scope: Begins in late infancy, extending into adolescence, with up to 50% of synapses pruned by adulthood to refine brain structure and function.
-
Removal of Redundant Connections: Weaker, unused neural connections are eliminated, focusing development on efficient and necessary pathways.
-
Role of Glial Cells: Astrocytes and microglia play a crucial role in mediating synaptic pruning, flagging unused synapses for removal.
-
Importance of Inhibitory Input: Inhibitory neural inputs also contribute to the pruning process, helping to refine synaptic strength and organization.
-
Reduction in Signal Overlap: The elimination of excess synapses reduces signal overlap, enhancing neural specificity and reducing gray matter density.
-
Restructuring Critical Pathways: Significant pruning occurs in areas like the visual cortex after critical developmental periods, and thalamocortical pathways are restructured to optimize brain function.
-
Streamlining Sensory and Association Areas: Synaptic pruning streamlines sensory processing and association areas, peaking then declining in language circuitry during childhood, while emotion and memory circuitry continues to consolidate into adulthood.
-
Enhancement of Neural Efficiency: The myelination of retained pathways post-pruning accelerates signaling, optimizing the computational efficiency of neural circuits.
-
Formation of Functional Modules: Pruning supports the formation of functional neural modules, indicating higher coordination in retained connectivity and stabilizing the brain's architecture for long-term efficiency.
-
Implications for Mental Health: Suboptimal pruning is implicated in various disorders, including schizophrenia and depression, highlighting its role in the "use it or lose it" process of neural selection.
-
Balance between Plasticity and Efficiency: The ongoing process of synaptic pruning maintains a balance between brain plasticity and computational efficiency, crucial for adaptive brain function and cognitive development.
Myelination (Starts late in gestation and continues into adulthood)
Myelination is the process by which myelin, a protective fatty substance, forms around the axons of neurons. This process helps increase the speed at which information travels through the nervous system.
-
Insulating Myelin Sheath: Myelination involves the formation of an insulating sheath around neuron axons, crucial for protecting and insulating nerve fibers.
-
Accelerates Neural Signals: Enables saltatory conduction, allowing electrical signals to jump from one node of Ranvier to the next, significantly accelerating signal transmission.
-
Early Onset: Myelination begins in subcortical regions as early as 5 months gestation, continuing robustly just before birth and peaking during early childhood.
-
Enhanced Communication Speed: Results in up to 10 times faster communication between neurons, facilitating rapid and efficient brain activity.
-
Supported by Glial Cells: Oligodendrocytes in the central nervous system and Schwann cells in the peripheral nervous system are specialized for this process.
-
Foundation for Complex Cognition: Essential for the development of complex cognitive abilities and skills, myelination lays the groundwork for future learning and memory.
-
Structural Composition: The myelin sheath is formed by the tight wrapping of glial cell membranes around axons, creating compact layers crucial for neural function.
-
Metabolic Support: Provides essential metabolic support to axons, ensuring their health and functionality.
-
Nodes of Ranvier: Gaps in the myelin sheath, known as nodes of Ranvier, are key for boosting signal transmission along axons.
-
Developmental Sequence: Myelination follows the sequence of neuronal connectivity, prioritizing sensory and motor pathways before advancing to more complex integrative brain regions.
-
Regional Differences: The timing of peak myelination acquisition varies across brain regions, with areas like the parietal, temporal, and frontal lobes continuing to myelinate into the early 20s.
-
Supports Integrated Brain Function: Facilitates the integration of various brain functions, supporting the brain's capacity for complex processing and learning.
-
Learning and Plasticity: Myelination contributes to the brain's plasticity, improving learning capacity and adaptability through adulthood.
-
Dynamic and Experience-Responsive: A highly dynamic process, myelination is responsive to neuronal activity and experiences, highlighting its role in brain development and adaptation.
-
Link to Developmental Disorders: Delays or abnormalities in myelination have been implicated in various developmental disorders, including autism, underscoring its importance for healthy brain development.
Reference
YouTube
|
|